Bone biomaterials for overcoming antimicrobial resistance: Advances in non-antibiotic antimicrobial approaches for regeneration of infected osseous tissue
Eliminating bacterial infection and simultaneously providing a bioactive environment for bone growth during the treatment of severe osteomyelitis is one of the greatest global challenges of modern orthopedics. Additionally, antibiotic resistance has been deemed a major threat to public health resulting in 700,000 deaths globally per year. Therefore, the development of multifunctional non-antibiotic antimicrobial biomaterials that could locally fight highly resistant bacteria while providing a template for osseous tissue ingrowth would be a major breakthrough. Current research focuses on a myriad of technologies to create non-antibiotic antimicrobial pro-osteogenic biomaterials, ranging from the inherently antimicrobial, based on the effects of chemistry or topography, to the application of antimicrobial metal ions and oxides, polymers, or peptides, as well as the potential of utilising biofilm degrading enzymes, quorum sensing drugs, and bacteriophages. In this review, they have provided an overview of the currently available treatments for osteomyelitis with a special focus on novel, non-antibiotic based solutions currently being developed and their antimicrobial mechanisms. Finally, they will present a discussion on future directions in the clinical translation of alternative biomaterial-based approaches to treat osteomyelitis.
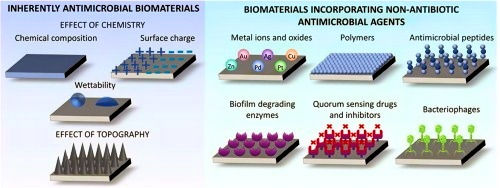
Bone infection, known as osteomyelitis, which occurs following microbial invasion or due to autoinflammatory processes, can drastically compromise the functionality and regenerative capacity of osseous tissues. These infections can involve single or multiple regions within the bone, and if not treated can spread throughout the body. Osteomyelitis can develop due to exogenous sources following a trauma or surgery, or secondary to vascular insufficiency which is seen primarily in patients with diabetes. Less commonly (estimated 20% of cases), osteomyelitis can also be caused endogenously via hematogenous spread which is seen primarily in young children and the elderly .

The World Health Organization and Centers for Disease Control and Prevention estimate that antibiotic-resistant, bacterial infections affect over 2 million people annually within the United-States alone. Bacterial resistance can be either inherent or acquired via mutations (alterations within the genetic code of the bacteria itself) or through horizontal gene transfers (plasmid-encoded resistance genes cross bacterial genera). The most common acquired resistance mechanisms are based on the minimization of intracellular concentration of antibiotics, modification of the antibiotic target through gene mutation, expulsion via efflux pomps, or the inactivation/degradation of the antibiotic. These mechanisms can be found in both gram-positive and gram-negative bacteria. The thick peptidoglycan wall in gram-positive bacteria (e.g. S. aureus) facilitates the adsorption of toxins or antimicrobials leading to slower development of resistance mechanisms, thus making gram-positive bacteria more prone to eradication. Instead, the wall of gram-negative bacteria (e.g. E. coli or P. aeruginosa) is composed of multiple peptidoglycan structures covered by an outer membrane containing toxins (lipopolysaccharide). This distinctive structure of gram-negative bacteria makes them more resistant to penetration by antimicrobial drugs, thus causing increased resistance and resulting in significant morbidity and mortality worldwide.
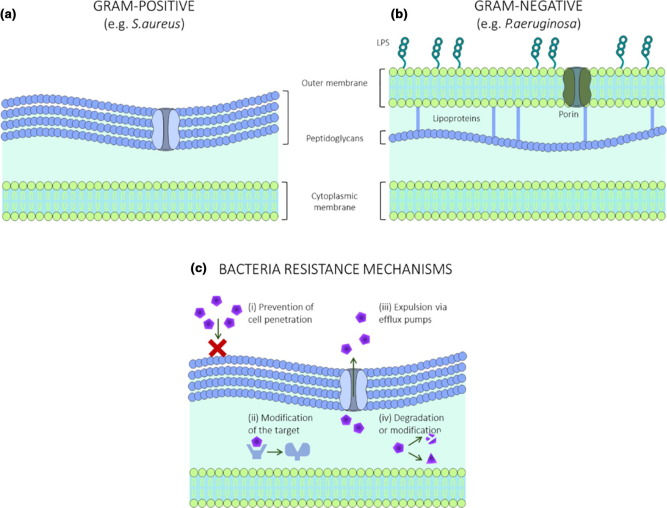
(a) Gram-positive cell envelop composition, (b) Gram-negative cell envelop composition, (c) Four commonly acquired bacteria resistance mechanisms: (I) reduced permeability of the cell wall and subsequently preventing antibiotic penetration, (ii) Modification or protection of the antibiotic target to prevent effective antibiotic binding, (iii) Increased efflux pump expulsion, and (iv) degradation or modification of the antibiotic within the bacteria through increased enzyme production.
Bone tissue engineering mainly relies on the combination of biomaterials, cells and bioactive molecules to repair, replace, or maintain damaged tissues. A wide range of materials, from biological and synthetic polymers, ceramics and composites have been adapted for bone regeneration. A number of considerations are required when developing biomaterials for tissue repair including biocompatibility, biodegradability, their mechanical properties, micro-and macro-architecture, and manufacturing scalability. Furthermore, they should not elicit a negative foreign body response within the host tissue and ideally, they should degrade at an optimal pace allowing the ingrowth of newly formed tissue. Furthermore, the stiffness, pore size, and porosity of the biomaterial should facilitate cellular infiltration and promote stem cell osteogenic differentiation. Lastly, it is crucial that the manufacturing and post-processing techniques applied are cost-effective and scalable such that the product is clinically and commercially viable.
In order to provide optimal osteointegration of a scaffold and enhance its osteoinductive and osteoconductive features, bioactive factors, are frequently incorporated within the biomaterial matrix to induce pro-osteogenic and/or pro-angiogenic functionality. Various strategies have explored the delivery of inorganic materials (hydroxyapatite, bioactive glass, etc.), nanomedicines, or the use of peptides and ECM components to further dictate the cellular response towards the optimal result in both the laboratory and the clinical field. Additionally, in the case of osteomyelitis, there is an obvious need to include antimicrobial agents alongside the osteogenic strategies to work synergistically and effectively regenerate bone while inhibiting bacterial growth.
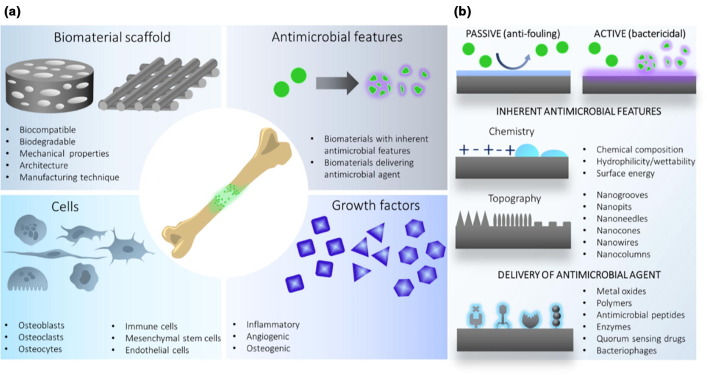
(a) Tissue engineering factors and design considerations for the regeneration of infected bone with biomaterials. Biomaterial scaffolds much are biocompatible, biodegradable, with appropriate mechanical properties and architectures while also easily manufactured. The scaffold should eradicate bacterial infection either through its inherent antimicrobial properties or via the delivery of antimicrobial agents. Furthermore, it should create positive feedback with cells involved in the bone healing process supporting its growth and infiltration. Some biomaterials might also deliver growth factors to enhance its angiogenic and osteogenic action. (b) The antimicrobial action of biomaterial could be either passive (anti-fouling) or active (bactericidal). This can be achieved either through modulation of physicochemical features of biomaterial or by the incorporation of an antimicrobial agent.
The grafting of biomaterials with antimicrobial molecules arises as an alternative strategy when bone grafts do not offer inherent antimicrobial features. These systems allow local delivery of antimicrobials which is beneficial as, unlike systematic administration, they do require high doses of active agents. The biomaterials can be used as a platform for the delivery of non-antibiotic agents including metal oxides, polymer coatings, peptides, biofilm-degrading molecules, bacteriophages, etc.
There are several approaches for the incorporation of antimicrobial molecules into biomaterial including i) physisorption/coating of the surface of the substrate (e.g. metals, ceramics), ii) internal encapsulation/physical entrapment through the biomaterials’ matrix (e.g. hydrogels), or iii) chemical immobilization/functionalization of scaffolds (e.g. metals, hydrogels) with an antimicrobial. All strategies share the common goal of eradicating bacterial infection through controlled and prolonged delivery of antimicrobial molecules.
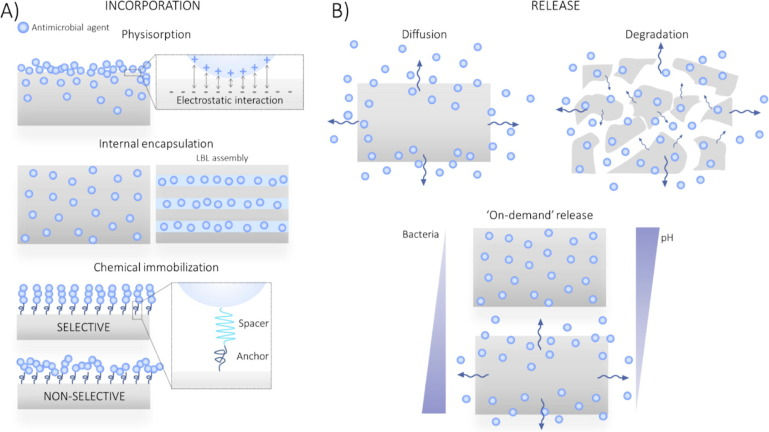
The incorporation of antimicrobial agents has been a frequently applied strategy in the development of bone biomaterials with anti-fouling or bactericidal features. Among them, the local delivery of antibiotics from biomaterials has been an approach that has shown success clinically with commercially available products (e.g., Stimulant (Biocomposites), Genta-Coll (Resorba), Septocoll E (Biomet UK Ltd., etc.). However, the evolving problem of antimicrobial resistance has shifted the interest of researchers and clinicians into utilizing non-antibiotic therapeutics such as metal ions, polymers, or antimicrobial peptides (AMPs). The incorporation of these potential non-antibiotic antimicrobial therapeutics into bone biomaterials might offer a potential multifunctional platform to support the growth of osseous tissue while eradicating resistant strains of bacteria. However, one should remember that while non-antibiotic approaches are a potential solution for antibiotic-resistant bacteria, some strains can evolve mutations against even these therapies, leading to the development of bacterial resistance mechanisms.
The antimicrobial properties of metal have been used as a water disinfectant, food preservation, and in medicine going back multiple millennia. An ancient Egyptian medical text, the Edwin Smith Papyrus, first documents the use of copper salts to prevent infection in burn wounds, while silver sutures have been used historically to repair vaginal tears following childbirth. Due to their inherent antibacterial properties, copper, silver, and zinc are promising alternatives to the use of antibiotics in bioactive materials.
The bactericidal effects of metal-based antimicrobial (MBA) particles are dictated by many factors including size, shape, and surface charge as well as intrinsic features of the bacterium against which they are used. The most widely accepted mechanisms of metal toxicity towards bacteria are the production of reactive oxygen species (ROS), protein dysfunction, impairment of membrane function, genotoxicity, and interference with nutrient uptake. ROS are highly cytotoxic and reactive molecules that are formed by incomplete reduction of oxygen, and the oxidative stress occurs from an imbalance in ROS homeostasis leading to protein and DNA damage. ROS often triggers other toxicity mechanisms since oxidation of amino acid side chains in proteins has been shown to provoke protein degradation and dysfunctionon. It has also been shown that certain metals, including silver, target the bacterial membrane to exert toxicity, as visually shown through electron microscopy of S. aureus and E. coli which highlights the membrane morphology disruption and leakage. Overall, it has been hypothesized that there is often not just one mechanism at work to cause bacterial toxicity and that the combination of various effects together results in cell death which makes the use of MBAs as non-antibiotic antimicrobial agents a promising alternative for biomaterials for bone infection1.
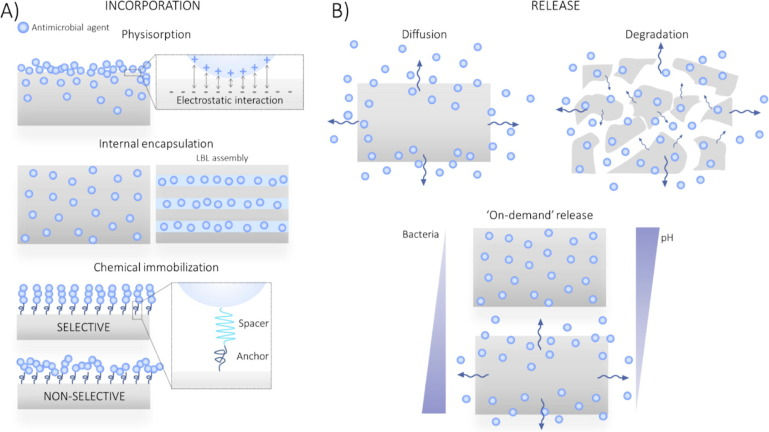
(a) Antibacterial mechanisms of metal toxicity. (b) The incorporation of cooper-doped bioglass into collagen (CuBG) scaffold significantly decreased the growth of S. aureus and enhanced the mineralization. Adapted and reproduced with permission. Copyright © 2019 Elsevier Ltd. (c) Mechanisms of action of membrane-active antimicrobial peptides (AMPs). Adapted and reproduced with permission. Copyright © 2005, Springer Nature. (d) The combinational strategy based on the incorporation of antifouling polyethylene glycol (PEG) layer and peptidic platform onto titanium substrate significantly reduced the adhesion of S. sanguinis while supporting adhesion and spreading of osteoblasts. Adapted and reproduced with permission
Joanna M. Sadowska, Katelyn J. Genoud, Daniel J. Kelly, Fergal J. O'Brien,
Bone biomaterials for overcoming antimicrobial resistance: Advances in non-antibiotic antimicrobial approaches for regeneration of infected osseous tissue,
Materials Today,
2021,
ISSN 1369-7021,
Comments