3D-printed gelatin methacrylate (GelMA)/silanated silica scaffold assisted by two-stage cooling system for hard tissue regeneration
Among many biomaterials, gelatin methacrylate (GelMA), a photocurable protein, has been widely used in the 3D bioprinting process owing to its excellent cellular responses, biocompatibility, and biodegradability. However, GelMA still shows low processability due to the severe temperature dependence of viscosity. To overcome this obstacle, they propose a two-stage temperature control system to effectively control the viscosity of GelMA. To optimize the process conditions, they evaluated the temperature of the cooling system (jacket and stage). Using the established system, three GelMA scaffolds were fabricated in which different concentrations (0, 3, and 10 wt%) of silanated silica particles were embedded. To evaluate the performances of the prepared scaffolds suitable for hard tissue regeneration, they analyzed the physical (viscoelasticity, surface roughness, compressive modulus, and wettability) and biological (human mesenchymal stem cells growth, western blotting, and osteogenic differentiation) properties. Consequently, the composite scaffold with greater silica contents (10 wt%) showed enhanced physical and biological performances including mechanical strength, cell initial attachment, cell proliferation, and osteogenic differentiation compared with those of the controls. Their results indicate that the GelMA/silanated silica composite scaffold can be potentially used for hard tissue regeneration.
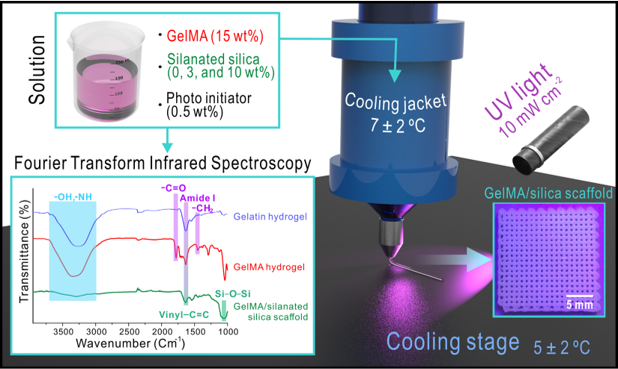
A scaffold is a biomaterial alternative that structurally supports the damaged areas of the internal body and serves a role as an artificial tissue and organ or a prosthesis. The scaffold should replace the tissue or organ directly proportional to their maturation rate. Therefore, the scaffold structure must possess the mechanical properties required to perform their role while being coexisted with the host tissues within the body, and the biological properties similar to that of the human body to induce tissue growth and maturation.
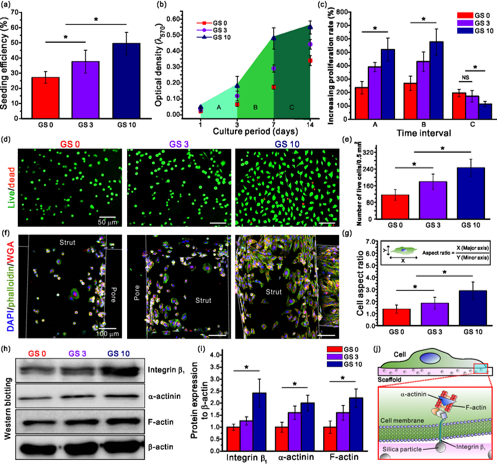
(a) Cell seeding efficiency of the hMSCs on the scaffolds after 4 h. (b) Cell proliferation results for 1, 3, 7 and 14 days and (c) the cell proliferation rate at different time intervals (A, B and C). (d) Live/dead images of the scaffolds after 24 h and the corresponding (e) results of the viable cell number. (f) DAPI/phalloidin/WGA images after 24 h and (g) the cell aspect ratio results. (h, i) Relative protein expression of integrin β1, α-actinin and F-actin. (j) Schematic showing the mechanism of signaling pathway.
In general, biodegradable polymers are used as the main ingredient to fabricate the cell carrier. Biodegradable polymers can be grouped into two categories: synthetically and naturally derived polymers. Especially, there has been an increasing number of reports that utilize protein-based hydrogels (collagen, alginate, chitosan, silk-fibroin, and gelatin, etc.) that have similar characteristics to native tissues to improve bio-functionality, such as cell culture, proliferation, and differentiation.
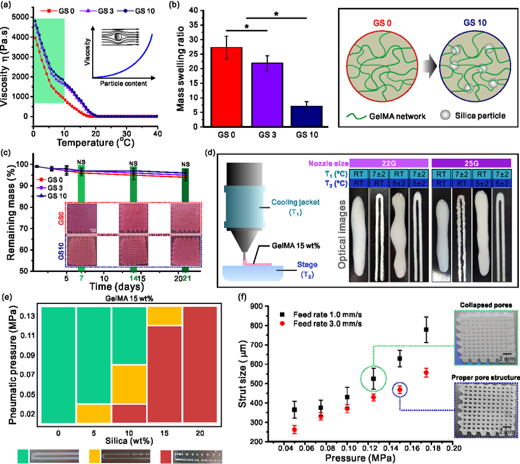
(a) Rheological, (b) swelling and (c) degradation test results of the scaffolds. Printability depending on (d) temperature of the jacket and stage and (e) applied pressure and silica content. (f) Strut size depending on the feed rate and applied pressure.
Protein-based hydrogels are widely used as bioink materials for 3D bioprinting due to the suitable characteristics of protein (biodegradability, biocompatibility, and cytophilicity). Recently, there are many ongoing studies on the utilization of protein-based 3D scaffolds necessary to regenerate organs, such as bone and cartilage. For example, gelatin is a natural polymer in a single-helix form derived by denaturation of triple-helix structured collagen that constitutes the skin, bone, and ligament of animals. Gelatin is easily dissolved in water and acetic acid, and due to its excellent biocompatibility, it is widely used in the field of tissue engineering. Since it has a similar composition as collagen, it is often used for the regeneration of bones and cartilages. However, gelatin, which has excellent biocompatibility, is not suitable for use in bone tissue regeneration because of its thermal degradation at body temperature (37°C). To address this issue, there have been efforts in overcoming the rapid degradation of gelatin by using chemical crosslinkers such as genipin or glutaraldehyde. However, due to the toxicity caused by the chemical crosslinking process, biocompatibility can be compromised.

Gelatin can be functionalized into gelatin methacrylate (GelMA) by introducing a methacrylate group in which the mechanical strength can be improved through a photo-crosslinking process. Therefore, it has been used not only in bone regeneration but also in the regeneration of many different tissues. In a study by Byambaa et al., a bone-like tissue structure was fabricated composed of GelMA using an extrusion-based 3D printing method. The fabricated hollow vascular structure was seeded with human umbilical vein endothelial cells (HUVECs)/mesenchymal stem cells (MSCs) and cultured for 21 days. As a result, high cell viability and a successful formation of bone and vascular structures were achieved. However, rapid decomposition of the structure due to the low degree of methacrylation affected the mechanical structural stability and posed an issue in maintaining the bone tissue structure for 21 days.
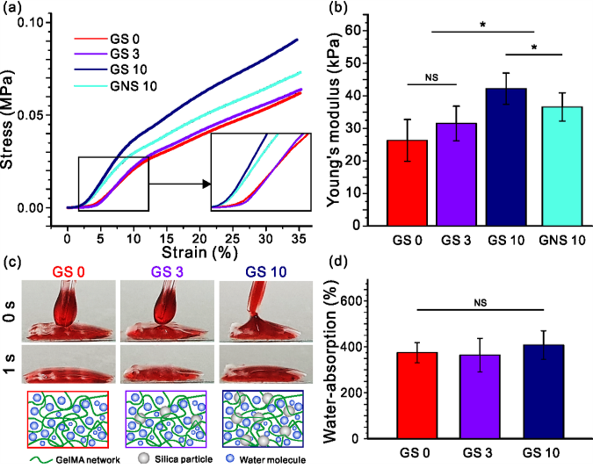
(a) Stress–strain curves and (b) Young’s modulus results of the scaffolds. (c) Wettability and (d) water absorption ability results of the scaffolds.
In another study, nanosilicates were incorporated to enhance the physical and biological properties of GelMA bioink. The addition of 2 wt% nanosilicates resulted in a fourfold increase in compressive modulus compared to that of pure GelMA. Furthermore, the incorporation of the nanosilicates significantly increased the viscosity of the GelMA solution at 37°C. The shear-thinning properties of the GelMA/nano silicate bioink enabled the printing of 3D structures. Finally, the nanosilicates enhanced cellular activities such as cell adhesion, proliferation, and osteogenic differentiation. However, the printing of high-resolution structures might be challenging. GelMA-based biomaterials for tissue engineering, especially applicable to bones, have shown shortcomings in mechanical properties, degradation rate, structure stability, as well as biological activities, which need improvements. Furthermore, for the clinical applications of GelMA, considerable studies on biological activities including osteoimmunology are necessary.

(a) ALP- and ARS-stained images of the scaffolds. Relative (b) ALP activity and (c) calcium deposition results. (d, e) Relative protein expression of BMP-2, collagen 1, osteocalcin and bone sialoprotein. (f) Fluorescence images of DAPI/WGA/osteopontin staining of the scaffolds.
Eunjeong Choi, Dongyun Kim, Donggu Kang, Gi Hoon Yang, Bongsu Jung, MyungGu Yeo, Min-Jeong Park, SangHyun An, KyoungHo Lee, Jun Sik Kim, Jong Chul Kim, Woonhyeok Jeong, Hye Hyun Yoo, Hojun Jeon, 3D-printed gelatin methacrylate (GelMA)/silanated silica scaffold assisted by two-stage cooling system for hard tissue regeneration, Regenerative Biomaterials, Volume 8, Issue 2, March 2021, rbab001, https://doi.org/10.1093/rb/rbab001
Comments